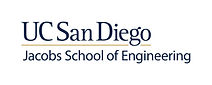
GROUP 23: CELL SORTING
ABOUT

OUR VISION
Clinical diagnostics and most modern biological research rely heavily on the study and isolation of subcategories of cells and molecules. Isolation of subcellular cultures allows for quick and accurate clinical diagnostics. Rapid and reliable isolation of cell subsets is the first step in any sort of analysis. We focus on the separation of cells in blood for diagnostic purposes.
​
Current cell sorters cost between $40-100,000 depending on quality and are often not very portable. Our design aims to be cheaper through the use of 3D printing manufacturing techniques and relatively portable.
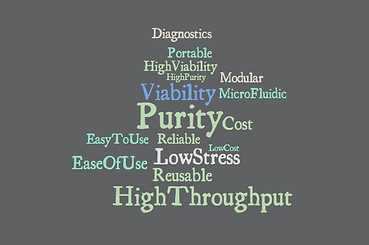

OUR STORY
With the help of the University of California, San Diego and their Bioengineering department, our team was able to further research the isolation of blood cells. Dr. Subramaniam, Chair of UC San Diego’s Bioengineering Department, was our leading principal investigator for the project. Our team consisted of a variety of Bioengineering majors, including Biotechnology, Bioengineering, and Biosystems.
Rachel Daniels is age 21 and majoring in Bioengineering: Biotechnology. Her background in fluid dynamics and bioprocessing was essential in the mathematical analysis and proessing. Rachel is most surprised about how much she learned during project design. She is now skilled in both AutoCAD and Comsol fluid dynamic processing. She has also learned how complicated cell sorting actually is and how difficult the task at hand may be. Rachel says, however, though something may seem impossible, it is always wise to seek help and advice in your work. Knowledge is most powerful when it is networked.
​
Aaron Patel is age 21 and majoring in Bioengineering: Biotechnology. His familiarity with cell morphologies and research into existing microfluidic devices was key to developing AutoCAD designs.
Aswini Krishnan is age 21 and majoring in Bioengineering: Bioengineering. Her background in her heavily mechanical engineering-based major has allowed her to gain significant experience in CAD design, which was applied to this project. While working on this project, Aswini enjoyed learning more about Solidworks computational fluid dynamics analyses to test the microfluidic chip design. As an aspiring physician-scientist, she has found great fulfillment in working on this project, which is aimed to provide a novel and low-cost diagnostic tool to make disease diagnostics more accessible in third-world countries.
Prarthana is age 21 and majoring in Bioengineering: Biosystems. Her background in usage of computational algorithms for engineering analysis helped her computationally test properties of the microfluidic chip. Through the course of the project, she enjoyed learning how to use Solidworks for design of the chip.
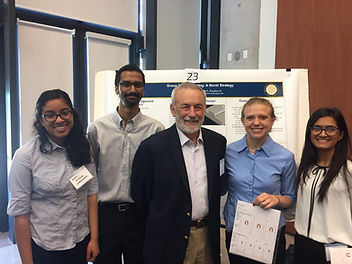
Background
Cell Sorting Methods
Current cell sorting technologies are split into two categories, bulk and single cell sorters. Bulk sorters are typically simpler than single cell sorters, and often use a single step to sort cells. Bulk sorters often look at a single cell characteristic to isolate cells into unique subsets. Due to this simplicity, bulk sorters typically sort large numbers of cells at high rates, however lack in cell recovery and purity. Current bulk sorting methods include centrifugation, filtration, affinity, and cell culturing.
​
The most prominent method of single-cell sorting is FACS, or Fluorescence Activated Cell Sorting, in which the forward, backward, and side scatter of light is used to determine the identity of the cell. Individual cells are first encased in droplets, and then sorted on the basis of their mechanical or electrical properties. The development of fluorescent-tagged antibodies has also improved the purity and recovery rate of FACS. By directing these fluorescent-labelled antibodies to their specific molecules, intercellular as well as intracellular characteristics can be used to sort cells. Once cells are identified, they may be separated using either mechanic or electrical means. Electrical separation occurs by charging the identified cell which may then be pulled by charged plates to its corresponding side. Mechanical separation depends on a variety of bulky actions, such as streamlining, in order to sort cells. While the most commonly used due to high purity rates, drawbacks include low throughput and loss of cell viability due to high hydrodynamic stress.
​
The advent of microfluidic droplet sorting marks a huge step forward in cell sorting technologies. Because microfluidic devices function at biological scales, they are miniaturized, and so they are easily transportable, cost less to produce than traditional instruments used in FACS, and have higher processing speeds. Microfluidic sorters have the ability to characterize cells as well as compounds secreted by them, elucidating potentially unknown cellular interactions. Sorting parameters directed at both intracellular and extracellular compounds within the droplet allow for higher purity of recovered cells1. Research is currently aiming to further develop speed, purity, recovery, and portability. Further advances in cell sorting will not only greatly impact the research field, but also the ability to reliably treat and diagnose in the clinic
​
Rachel Daniels
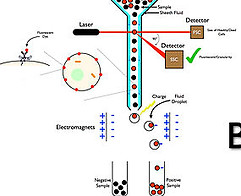

Microfluidic Device Design: Process Workflow
​
A three-stage design proposal with the goal of separating leukocyte subpopulations was formulated according to the workflow shown to the right. This year, the design and simulation stages were completed in which one phase of the chip was aimed at red blood cell (RBC) removal while the second phase served to isolate lymphocytes.
​
Due to unforeseen complications that arose in planning for fabrication, it was decided that printing the chip would be the following year's goal. This would be possible using the Nano3 facility following a PDMS molding and nanofabrication training session.
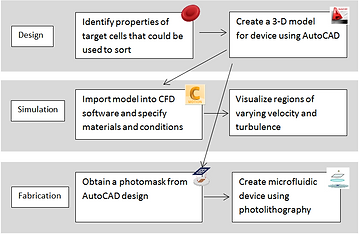
OUR SOLUTION
· High Purity · High Cell Viability · Low stress inducer · Sterile · High throughput · Reusable · Portable · Low cost · Modular
Our design aims to create a 3D-printed low-cost, reusable, and portable microfluidic chip that can achieve sorting of blood cells with 90% purity.
-
3D printing technologies allow for a cheap and reusable alternative to current cell sorting methods.
-
The microfluidics behind the design allows for easy portability and only a small volume requirement of blood.
-
Assuring a 90% accuracy is extremely important in diagnostics to ensure proper treatment is followed.
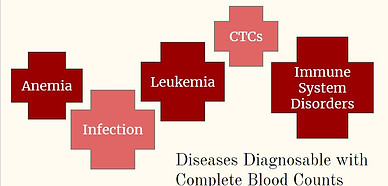
The over all layout of our chip utilizes several steps, or phases, for the isolation of each cell type. Space is conserved through the use of bifurcating channels and layers to separate flows.
​
We created two separate designs for accomplishment of our goals, with distinct strengths and weaknesses, to be tested in the wetlab after fabrication for better assessment of efficacy.
01 / Erthrocyte Separation
Erythrocytes (RBCs) outnumber leukocytes (WBCs) by a factor of 1000:1. Also, due to their singular function of transporting oxygen to various regions of the body, RBCs are of little interest to immunologists and clinicians. Therefore, the first phase of our chip is aimed at removing the majority of RBCs from blood plasma. This is accomplished by taking advantage the relatively largee concentration of iron in red blood cells and magnetic properties due to it. Our chip aims to pull oxidized red blood cells from the rest of the blood flow using a strong enough magnetic field. This push pull will end with a bifurcation channel in which the red blood cell free flow will continue down the chip for further isolation.
Both designs used these concepts for RBC separation. In the first design, a magnetic field will be initiated in the cell flow channel perpendicular to the direction of cell flow. As red blood cells have iron and therefore are magnetic, at the end of the channel they should theoretically flow in a straight stream near the magnet while the white blood cells will be redirected. This is shown in the picture below.
​
​
​
​
​
​
​
​
​
​
​
​
​
​
​
​
​
​
​
For the second design, Layer 3 is defined as the topmost layer, Layer 2 as the middle layer, and Layer 1 as the bottom layer (not the bottom of the chip, but rather the bottom CAD layer, as labeled in Figure 5. Cells enter the chip through the hole on Layer 3, and pass down to Layer 1 to the straight vertical channel. A magnet of 1T strength on the left side of the channel causes the iron-rich red blood cells to deviate toward the left side of the channel when passing through the channel, and exit the chip through the indicated outlet on the left side. All leukocytes, as they are not attracted to the magnet, flow through the channel on the right of the bifurcation into Phase 2.
​
​
​
​
​
​
​
​
​
​
​
​
​
​
​
​
​
​
​
​
​
​
We were able to computationally test fluid dynamics in our 3D CAD models. For Design 1, we used Comsol fluid simulation. Due to Blood non-newtonian state when running through capillary tubing, we decided to model the blood according to the Carreau model. The Carreau model has the highest viscosity in all shear rate ranges and the Newtonian model has the smallest viscosity in the significant shear rate ranges in our problem domain. The Carreau model may be seen below:
​
​
​
​
​
​
​
​
​
​
​
​
​
​
​
​
​
​
​
​
​
​
​
​
​
​
​
​
​
​
​
​
​
​
​
​
​
For Design 2, we opted to use the Solidworks Flow Simulation software to model velocity and pressures of fluid through two particular structures in our design: first, the 180 degree loop after RBC separation, and second, the dispersion of our cells into many different chambers for lymphocyte separation, due to their structural complexities that may result in high pressure, backflow, and potential mixing if not properly designed.
​
For the purposes of simulation, we simulated the blood according to the properties of water as we plan to dilute the blood before use in the chip, as the proof-of-concept wet lab experiments indicated that a 1:40 dilution seemed to work optimally for blood counts. Our analysis indicated that pressure decreased around the curve to match atmospheric pressure at the time of reaching the multi-channel component, suggesting that a drastic increase in pressure around the curve did not occur, and thus that significant stresses may not be placed on moving cells.
​
We also performed computational analyses to examine the velocity of fluid in the same parts, and found the initial velocity of the sample to be about 3mm/s, and the velocity around the turn to increase to about 6mm/s. Considering that our chip is ~20mm tall, this speed, would allow the fluid to travel through one direction of the chip in about 6 seconds, which is reasonable rate for our device. Interestingly, the higher velocity may be a reason for lower pressure because as per Bernoulli’s principle, higher velocity of fluid flow corresponds to lower pressure.
​
​
​
​
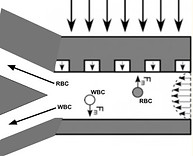

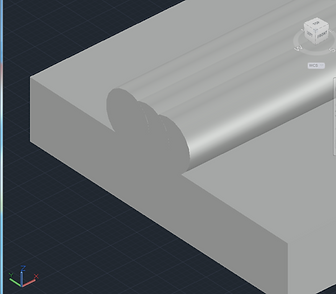
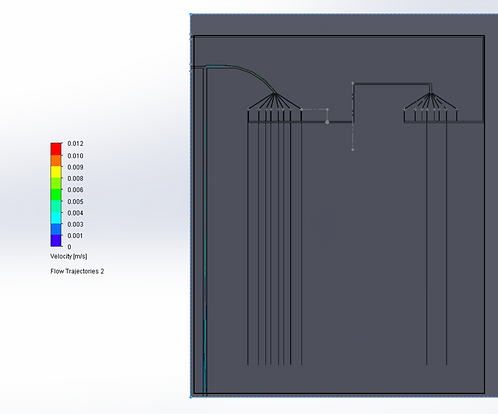
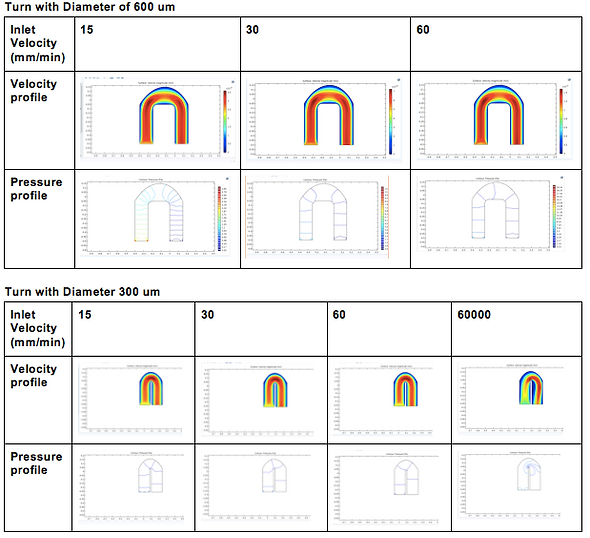

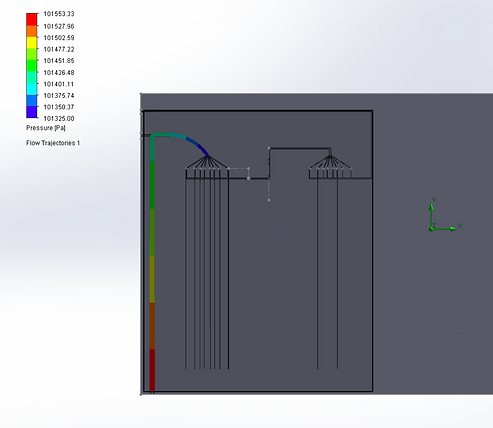
02 / Lymphocyte Separation
Lymphocyte separation would occur in the second layer following RBC removal. Due to the lymphocytes significant size difference when compared to other leukocytes, this phase focused on size exclusion methods. Features of this phase include several iterations of channels 10um in width designed to capture the lymphocytes but allow the larger leukocytes such as monocytes and neutrophils to continue flowing through the chip.
​
In our first design (below), due to the dramatic size difference between lymphocytes and the rest of the white blood cell population, sorting lymphocytes through a size filter seemed reasonable. Our design would include a section in which the lymphocytes pass through approximately 4 arrayed filters all 10 um in filter diameter size. Because printing the filter might present issues we proposed to insert filter into designated spot after printing.
​
​
In our second design (below), as mentioned previously, post removal of the erythrocytes, all remaining cells in the blood are forced to move to the right of the bifurcation in Layer 1, into the multi-channel component of our design. The dimensions of the fanning channels are 20uM, enough to accomodate all leukocytes, while the dimensions of the vertical channels that follow are 10uM width, only enough to accomodate lymphocytes. As a result, only lymphocytes can ultimately pass through the channels down the chip.
​
As a result, we expect clogging to occur near at the intersection of the fanning channels and the narrow channels due to inability of other cell types to pass down the narrow channels. To account for this, we designed a flushing system in order to remove clogging cells from the system. A PBS wash solution will be inserted and pushed by syringe through the downward tubing in Layer 3, to reach Layer 1, as indicated. Horizontal valves will prevent backflow of the leukocytes into the large arched channel, forcing them through the fanning channels and up the vertical channels into Layer 2. These cells will then be collected and carried along Layer 2, and then again go vertically downward to re-enter Layer 1. Another repetition of the same process is done, to account for lymphocytes that may have been flushed out to Layer 2 along with clogging cells. The number of repetitions to be done to achieve our 90% purity rate will need to be determined by testing a fabricated chip in the wet lab, and can easily be added to our design.
​
​
​
​
​
​

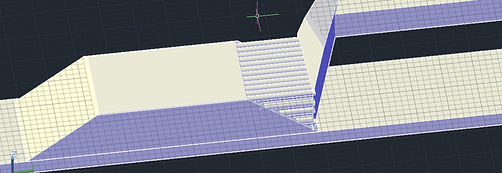
03 / Neutrophil Separation
One possible avenue for neutrophil isolation is Affinity Flow Fractionation (AFF), which relies on cell-specific ligands to pull target cells out of the flow. Nuetrophils have been shown to have a high affinity binding to p selectin ligands specifically. By printing small grooves capable of housing the p-selectin yet not hindering blood flow, we may dirct neutrophil flow specifially. This cell rolling effect channels our neutrophils without having an end bound product.
​
​
​
​
​
​
​
​
​
​
​
​
​
​
​
​
We expect both designs to achieve similar efficacy of separation for Phase I of the design, as both operate with a 1T magnet to have bulk-separation of erythrocytes from the rest of the cells in the blood. The primary differences in the chip are associated with Phase 2 of the separation, which involves lymphocyte sorting. A major pro of our first design is that it is aimed to achieve high throughput with an array of channels through which the blood cell mixture flows, while the second design, at the expense of high throughput, has narrow single-lymphocyte movement channels that will significantly impede movement. It also requires potentially several iterations of lymphocyte separation to achieve high accuracy of separating most lymphocytes from the mixture (90%). However, a pro of the second design is that it focuses on achieving accuracy in this way, while a con of the first design is that the accuracy may not be the expected 90%. The second design can have as many iterations of lymphocyte separation inserted into the chip design as needed to achieve a high accuracy, which may be much more difficult in the first design due to the array of channels making it difficult to implement an effective wash system that is necessary to remove unsorted cells, as we are able to do in the second design. Whether our first high throughput design will separate lymphocytes with high accuracy must be tested in the wet lab after fabrication of the chip, and the exact speed of our second design’s Phase 2 must also be determined in the wet lab.
​
​
Pros and Cons of Designs
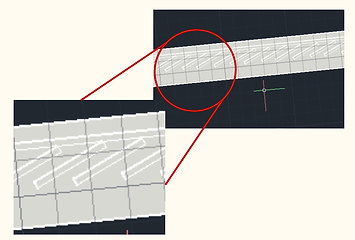
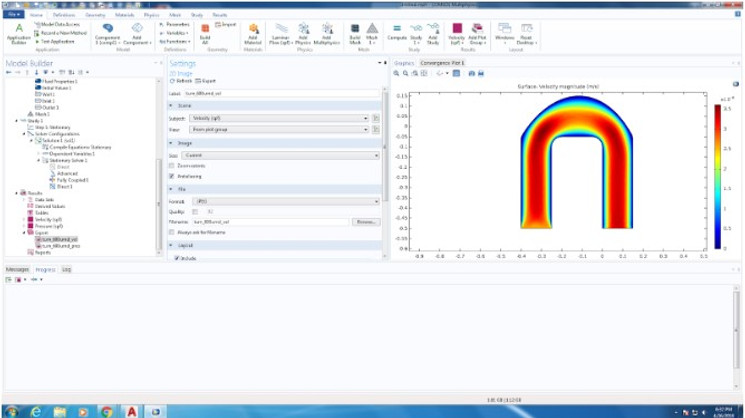
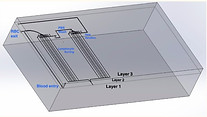
Aswini Krishnan
Ethical Considerations
​
In terms of our creation of the design, at this stage of the project, we opted to use rodent blood over human blood because of the additional safety risks associated with working with human blood. Upon further development of more realistic CAD simulations and subsequent microfluidic chip fabrication however, human blood can and should be used to test out the actual accuracy of the chip. For the purposes of our project at this point, we used all required PPE and followed required protocols while working with rat blood and disposed off all biohazardous materials appropriately.
​
Other safety considerations are risks associated with the tools we worked with. All pipette tips were disposed off as sharps, and special caution was exercised when working with the thin capillary tubes. Pipette tips that came in contact with rodent blood was disposed off as biohazardous materials. The magnets used for our experiments were below 0.5T, but still quite strong, and so care was taken so as to not place any magnetic materials or the magnets themselves in close proximity to each other.
​
With relation to the need of the user, our chip should be safe to use and pose little hazard to users, as the chip does not interact with the body for diagnoses but rather sorts a blood sample externally for diagnostic purposes. Potential safety issues include the removal of the blood sample, which should be done by a phlebotomist, and use of a sharp syringe to put the blood through the device. The user must be careful so as not to accidentally harm anyone through use of the syringe. No environmental issues should arise, as our chip does not create any toxins that will be released to the environment, and similarly no social issues should arise either as our chip does not serve a social function but rather addresses a medical need.
​
An ethical issue that may arise is whether a small sample of blood will be accurately representative of the whole blood population in the patient’s body when run through the chip to determine disease. As our chip can take a maximum volume rate of 5.87 * 10^-11 mm^3/s, it may be unlikely that a large amount of blood can be processed, and so it remains to be determined to what extent the test results can be clinically applicable, even if the test achieves high accuracy of sorting for the processed sample. Ethically, it is imperative to determine this so that clinically valid information is provided to patients.
​
The final microfluidic chip, when ready for mass production, must be made low cost and reusable as the goal of this project is to provide an affordable yet efficient means for blood cell sorting. Ensuring the reusability and durability of our device is important to evaluate to provide the customer with accurate information regarding their chip, in terms of marketing ethics.